Thermal beam clock
In this type of clock, a heated oven pierced with an orifice provided with channels generates a thermal atoms beam. We will only consider cesium thermal beams that are the most interesting for time metrology. The atoms, moving at few hundred meters per second, undergo various interactions as they move through a vacuum chamber.
Early generations of laboratory thermal beam clocks used magnetic deflection, analogous to the Stern and Gerlach experiment, for atomic state selection and clock signal detection. The such first clock was developed in 1955 at the National Physical Laboratory in England.
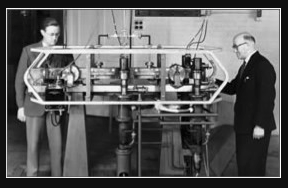
In the 1980s, this technique was replaced by laser interaction techniques allowing selection of the atomic state by optical pumping – a technique for which Alfred Kastler was awarded the Nobel Prize in Physics in 1966 – and the laser-induced fluorescence clock signal detection. In all thermal beam clocks, interaction between atoms and the signal generated by the oscillator takes place in a resonant cavity with a particular geometry called the Ramsey cavity (Norman Ramsey, American physicist, Nobel Prize in Physics 1987): the atoms undergo two successive interactions with the exciting field, these interactions being separated by a dead-time during which the atom state evolves freely without any interaction. Compared to a continuous interaction of the same duration, the Ramsey method makes it possible to refine the resonance line and to reduce some systematic effects influence.
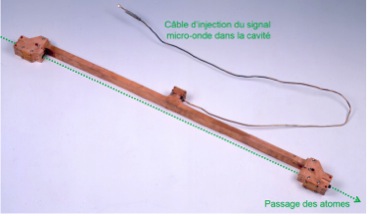
The best performances obtained on a cesium thermal beam clock optically laser pumped was obtained in the late 1990s at SYRTE and is around3.5×10-13 τ -1/2 for frequency stability and 6.4×10-15 for accuracy. These clocks are now surpassed in performance by cold atomic fountains, although several hundred commercial cesium thermal beam clocks of lesser performance are still sold worldwide each year.
Industrial versions of thermal beam atomic clocks have also been developed for ground or in space use. These clocks follow the same operating principle as the primary thermal beam standards described above, but their interaction cavity is much shorter (10-20 cm) in order to get a smaller device size.
The marketed thermal beam clocks today all use magnetic deflection for the clock signal preparation and detection. Thanks to their good long-term frequency stability, they are mainly used in metrology laboratories or large synchronization centers for timescales generation. As an indication, several hundred commercial cesium thermal beam clocks contribute today to the achievement of International Atomic Time (TAI). Some models have also been embedded in GNSS satellites. A direct relativistic time dilation measurement was also directly performed in 1971 with cesium clocks embedded in aircrafts.
The frequency stabilities of the best devices are better than10-11 for one second integration, with stability floors≤ 10-14. Their accuracy is5.10-13. As for the primary standards, the optical pumping magnetic selection replacement has shown its interest and new devices are being industry-developed for ground or space applications, with the objective of gaining one order of magnitude on the frequency stability.
Atomic resonator of a Cs clock with magnetic deflection (Thales Electron Devices-Oscilloquartz)
