Atomic clocks
The operating principle of an atomic clock is based on the oscillator frequency servo control on an absolute frequency reference corresponding to the transition frequency between two quantum states of an atom, an ion or a molecule. The clock’s useful signal is generated by an oscillator whose type depends on the considered frequency range.
The FIRST-TF members carry out numerous R&D activities on a wide variety of atomic clocks, differing in the reference atomic species (atom, ion, etc.), the reference transition frequency range (microwave, optics), the atomic source type (thermal beam, vapor, laser-cooled atoms, etc.), the performance class (stability, accuracy), the constraints (mass, consumption, volume, environmental resistance) related to the applications range, etc.
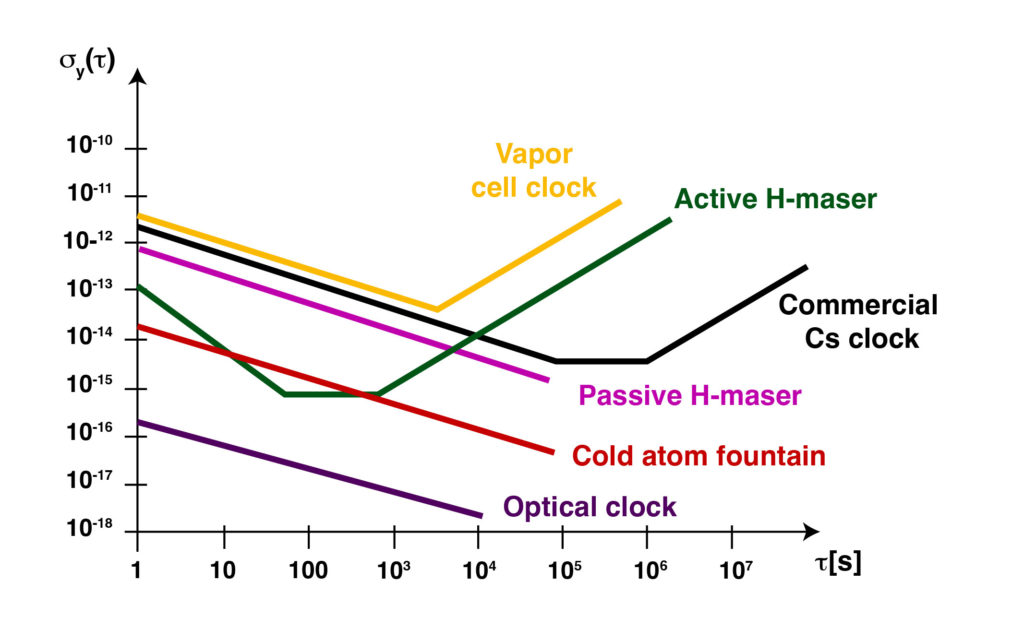
MICROWAVE CLOCK
Thermal beam clocks (FIRST-TF → SYRTE, Thales Electron Devices, Oscilloquartz)
Cold atom fountains (FIRST-TF → SYRTE, LKB)
Cold space atom clocks – the ACES/PHARAO mission (FIRST-TF → SYRTE, LKB, Sodern, Thales Electron Devices, CNES)
Compact cold atom clocks (FIRST-TF → SYRTE, LKB, µQuanS, TRT, CNES)
Coherent Population Trapping (CPT) clocks (FIRST-TF → SYRTE, FEMTO-ST, Syrlinks, Tronics)
OPTICAL CLOCKS
Ion clocks (FIRST-TF → PIIM, FEMTO-ST)
Clocks with trapped neutral atoms in optical networks (FIRST-TF → SYRTE)
Other developments (FIRST-TF → LKB)
In these clocks, the atomic frequency reference is in the optical domain: its value is four to five orders of magnitude higher than for a microwave clock. Even if the optical clocks frequency stability is still limited today by the insufficient spectral purity of the probing laser (via the so-called Dick effect), the best current optical clocks display frequency stabilities at the level of some 10-16 for an integration time of 1 second, which is 2 orders of magnitude beyond the best cold atom fountains operating in the microwave domain and whose frequency stability is in the range of 10-14 (at 1 second).
Similarly, the amplitudes of many systematic effects (Zeeman effect, collisional shift, etc.) that limit the atomic clock accuracy do not depend on the transition frequency value. Consequently, these effects will induce a relative frequency shift that is all the lower as the clock frequency is high. Thus, even if some systematic effects will remain the same in relative value – such as the effects related to the Doppler effect – a considerable improvement in the clock accuracy is obtained by moving from the microwave domain to the optical domain. Note that in this context, “accuracy” means the clock frequency error with respect to its reference atomic transition and not with respect to the current definition of the SI second, based on the Cs atom.
Two optical clocks types are currently being developed: ion clocks and trapped neutral atom clocks in optical networks. Choosing an atom or an ion among many elements that can be used in an optical clock result from a compromise between the considered element abundance, the frequency stability it may provide (resonance linewidth, signal-to-noise ratio, Dick effect degradation, …), the frequency accuracy it may provide (Doppler effect, light-shift, black body radiation shift, …) and the availability of reliable laser sources for probing, cooling and trapping. Today, these two pathways lead to ultimate frequency uncertainties at substantially the same level (in the range of 10-18 in relative value), i.e., more than 2 orders of magnitude beyond the cold atom fountains (whose accuracy is in the10-16 range).
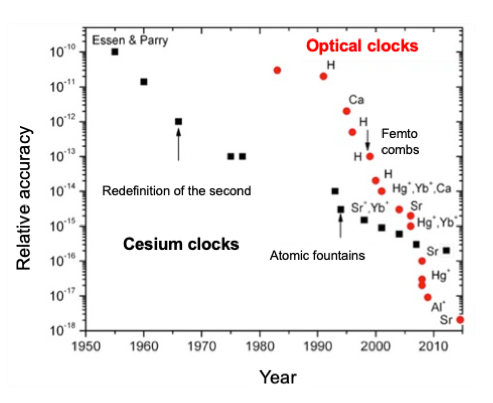