GNSS
Text inspired by the impact study of a GNSS alteration carried out by FDC
What is GNSS ?
Operating principles
1. Global Navigation Satellite System (GNSS) components
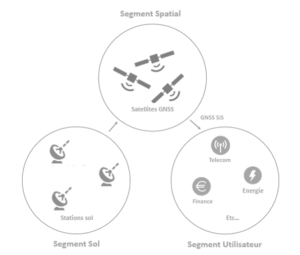
A GNSS system consists of three segments as shown in Figure 1 below.
- The space segment refers to the satellite constellation that continuously transmit distance measurement signals in space (SiS);
- The ground segment tracks the satellites and regularly transfers information on their positions and corrections to their atomic clocks (connection of each satellite clock to the global time scale of the GNSS constellation). The satellite positioning information is then time-tagged and transmitted to users in the form of “navigation messages” integrated into the SiS;
- The user segment refers to the receivers that use the signals sent by the satellites (SiS) to calculate their Position, Velocity and Time (PVT) data.
2. Principle of positioning and time estimation by GNSS
GNSS positioning is based on the principle of triangulation, which is relies on an estimate of the distances separating the receiver from each of the visible satellites. These distances are obtained from the calculation of the time separating the emission signal from its reception, knowing that the signal propagates at the speed of light. To calculate his position, the user must therefore also know the GNSS time used by the satellites.
A GNSS measurement therefore consists of calculating four variables: the three position variable of the receiver plus the time difference between the receiver’s reference and the satellite’s onboard clock. A minimum of four satellites are therefore required to perform a GNSS measurement.
Note that if we consider that the difference between the time reference of the satellite constellation and that of the receiver is known, three satellites are sufficient to deduce the position of the user. Even if in all generality three spheres intercept each other at two distinct points, one of the solutions can always be invalidated because the position or velocity associated with it can be considered as aberrant (not positioned on the Earth’s surface, etc.). The figure below illustrates the principle of triangulation applied to GNSS.
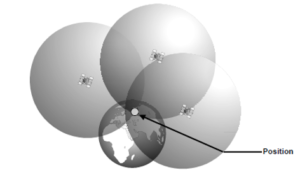
GNSS satellites transmit two types of data: PRN (Pseudo Random Noise) codes and navigation messages. PRN codes are used to calculate the receiver-satellite distance and to identify the satellites. The same receiver is thus able to receive signals from different satellites. The navigation messages contain various information such as the position of the transmitting satellite, its status, atmospheric data, and the parameter for connecting the satellite clock to the global time scale of the GNSS constellation. This information is used in particular to correct certain errors affecting the calculation of the geometric distance covered by a signal between a satellite and the receiver.
The position of the satellites must be continuously monitored to ensure the accuracy of the GNSS measurement. To do this, the satellites’ orbits are continuously monitored by the ground segment. Each satellite therefore receives frequent corrections to its estimated position, which it incorporates into the navigation message.
Determining the travel time of the wave with accuracy is also essential because, as its propagation speed is close to that of light, the slightest error in this measurement leads to a significant uncertainty in the calculated receiver-satellite distance. For example, an error of one microsecond in the travel time measurement leads to an error of 300 m in the calculation of the distance covered by the signal.
To ensure an adequate level of accuracy in the range measurements, the receiver first generates the codes transmitted by each of the satellites being tracked, these binary codes being predefined and specific to each satellite. Inter-correlation between the receiver-generated code and the code received from the satellites allows the receiver to determine the exact moment of arrival of the signal in its own reference frame. Using this information, and correcting for geometric distance and other sources of error, the receiver can deduce, the time difference between its internal time reference and the satellite clock or constellation time scale.
Thus, the accuracy of the delay measurement also depends on the quality of the correlation between the codes generated by the receiver and the corresponding codes transmitted by the satellites (see Figure 3).
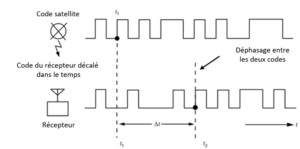
Determining the exact position of the satellites is also difficult since the satellites follow a quasi-elliptical trajectory. Thus, it is also necessary to continuously correct their position estimates to ensure the required accuracy of the receiver’s position calculation. Each satellite therefore frequently receives a correction to its estimated position, which it incorporates into the “navigation message” sent to the user.
3. Main existing systems
GNSS signals can be provided by multiple systems, including global or regional constellations and Satellite-Based Augmentation Systems (SBAS) providing correction broadcast via geostationary satellites.
The current global constellations are as follows:
- GPS: The first GNSS, fully operational since 1995, is managed by the US Department of Defense. It is currently being modernized with the deployment of new GPS III satellites;
- GLONASS: The Russian GNSS, fully operational since 2011, is managed by the Russian Aerospace Defense Forces. The latest generation of satellites, GLONASS-K entered service in February 2016;
- Galileo: This is the European GNSS, currently being deployed. In addition to the high-quality open service based on signals in the E1 and E5 bands, Galileo is also the first GNSS constellation to provide a return link for users in distress. Galileo also offers other unique capabilities such as an authentication function for the navigation message (OS-NMA) as well as an encrypted navigation signal implemented in a commercial authentication service (CAS). OS-NMA and CAS represent the first decoy protections available to all civilian GNSS users. Galileo will also provide free access to a high accuracy service, the HAS (High Accuracy Service). Finally, Galileo includes a regulated service, the PRS (Public Regulated Service), reserved for users authorized by governments for sensitive applications requiring a high level of service continuity;
- BeiDou (Phase 3) or BDS-3: The Chinese GNSS is currently being deployed to replace the current regional system. BeiDou is managed by the Chinese Navigation Satellite Bureau. Together with the regional positioning system, BeiDou is now the largest satellite constellation.

In addition to these global constellations, there are several augmentation systems that increase the accuracy of GNSS information. Among these systems, SBAS (Satellite Based Augmentation Systems) deliver real-time corrections to increase the accuracy of GNSS measurements while ensuring their integrity. Initially developed in the 1990s to introduce GPS in the civil aviation sector, their use is now being extended to many other sectors such as railways. There are currently four operational SBAS (WAAS in the USA, MASA in Japan, GAGAN in India and EGNOS in Europe) and five additional SBABs under development (SDCM in Russia, BDSAS in China, A-SBAS for ASECNA, KASS in South Korea and SPAN in Australia and New Zealand).
The following figure represents these different systems and their service areas:
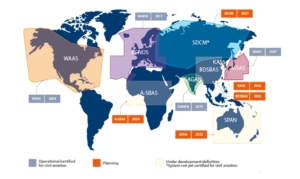
The main characteristics of SBAS systems are given in the table below:

Uses of GNSS
1. Time synchronization
The use of satellite systems (GNSS) for time synchronization applications has become essential in many sectors of activity. For example, it is currently used for :
- Generate time scales (especially UTC);
- Synchronize the clocks of the various elements of a single telecommunication network in order to transmit a data flow (voice, video, etc.) in an optimal manner;
- Synchronize the clocks of an ensemble of measuring devices in order to synchronize the measurements;
- Time-tag security certificates and check their validity;
- Synchronize the time-tagging of information received by data servers on the same computer network;
- Synchronize security systems, particularly for transportation systems;
- Conducting scientific experiments.
GNSS synchronization therefore plays a central role in many applications and sectors.
As GNSS can be used to provide a common time reference for equipment sharing a common network, it enables both:
- Cost savings: it is no longer necessary to distribute a time reference through dedicated physical channels (optical fibres, coaxial cables) which can be very costly to install and maintain for the operator;
- Simple implementation: GNSS receivers operate independently. Distributing GNSS receivers within a network may appear simpler and faster for operators than setting up a dedicated synchronization network;
- Access to satisfactory performance that meets the precision requirements of the most demanding applications and services.
More generally, in addition to being an essential element for the proper functioning of a single network, time synchronization is also used to interface between networks. GNSS signals have indeed become an essential, global and standardized means of disseminating a common time source for network operators.
All of these factors have driven the adoption of GNSS by network operators for time synchronization, to the point where GNSS has become a single point of failure for some applications.
2. Geolocation
With the reduction in size and cost of GNSS receivers, their use in the transportation sector is growing and diversifying. GNSS is used in the following areas in particular:
- Road transportation: GNSS geolocation is used in the field of road transportation for many applications such as navigation, fleet management, regulatory applications (tachograph, etc.), road safety, tracking of dangerous products or payment of road tolls;
- Intelligent and autonomous vehicles: as passenger vehicles become more and more intelligent and autonomous, these new functions may require the use of GNSS geolocation systems. These functions include communication and data exchange between vehicles/infrastructures/pedestrians (V2V, V2I, V2X), autonomous navigation, emergency calls and messages (eCall) or “Pay-as-you-drive” insurance;
- Rail: rail traffic management methods are evolving and GNSS is an integral part of these developments. Indeed, Europe is implementing new solutions for train management and signaling, notably through the development of the European Rail Traffic Management System (ERTMS), which is pushing for the integration of GNSS geolocation solutions for safety-critical applications;
- Maritime and Inland Waterway: The maritime transport sector is increasingly relying on GNSS geolocation for navigation at sea, near the coast and on inland waterways. The Automatic Identification System (AIS) relies on GNSS positioning of the vessel and GNSS is also used for the synchronization of the AIS system itself;
- Aviation: GNSS is used for both landing and take-off phases (e.g. LPV200 approaches) and in-flight navigation. The SESAR research program, a pillar of the Single European Sky, as well as the ICAO roadmap promote the use of GNSS geolocation for future air traffic control systems. GNSS could be used to enable continuous descent and ascent, reduced separation between aircraft and route network optimization.
In addition to these five main areas, new applications based on the use of drones are about to or have already entered the world of transport. These applications include delivery drones (already used for parcel or medicine delivery) and taxi drones (currently being tested). Whether it is to transport a parcel or a person, a drone today relies heavily on GNSS geolocation to determine its position and follow its flight plan. In addition, a set of services called U-Space Blueprint is being studied by SESAR in order to allow a safe integration of UAVs in the airspace, in particular through the implementation of a UAV traffic control system. The use of GNSS geolocation is particularly envisaged for such systems.
Ensuring the accuracy, integrity, continuity and availability of the GNSS geolocation service has become a major issue in the transportation industry.
[3] https://www.gps.gov/systems/gps/performance/accuracy/
[4] https://www.researchgate.net/publication/228936470_GNSS_Status_and_perspective#pf3
[5] https://www.gsc-europa.eu/sites/default/files/sites/all/files/Galileo-OS-SDD_v1.1.pdf
[6] https://gssc.esa.int/navipedia/index.php/Galileo_Performances#cite_note-Galileo_OS_SDD-1
[7] https://directory.eoportal.org/web/eoportal/satellite-missions/content/-/article/cnss
[8] http://www.csno-tarc.cn/en/system/introduction